Flywheels and other energy storage systems have the potential to change the way electric utilities operate. A while ago I put down my thoughts on flywheels in a 2010 article I share below in this blog.
The context for my thoughts is that the large central station model for utilities is changing as we move toward more decentralized power generation (think renewables). People are also beginning to react to the vulnerability of the current system to outages, whether accidental or deliberate, that leave thousands of people without power for extended periods of time.
Storage of energy, whether electrical or thermal, can reduce this vulnerability and allow greater use of variable (intermittent) renewable energy sources such as solar and wind. Pumped hydro, compressed air energy storage, and batteries have received the most attention to date. For batteries the major barriers have been insufficient storage capacity, purchase and maintenance costs, space requirements, and the inconvenience of replacement of heavy batteries.
Lead acid batteries have been used in cars, boats, buoys, aircraft and other applications requiring portable electricity sources for many years, and will be used for many years into the future. An interesting aspect of lead acid battery use was their powering of electrical vehicles in the early decades of the 20th century when electric vehicles were the dominant form of personal transportation. In fact, Mrs. Henry Ford drove an electric vehicle. This situation changed because of limited range then available with existing batteries and the advent of high energy density liquid petroleum fuels.
This may be changing today with the emergence of more energy dense and lighter lithium ion battery technologies, but cost is still a major consideration. While cheaper lithium ion cells are coming and lithium ion battery packs are being explored actively for a wide range of applications, including electric vehicles and utility power storage, I would like to suggest that flywheels may also have a role to play in our electric utilities’ future. This idea has been swirling around in my head for many years, and has been mentioned by others, but with the advent of advanced flywheels in recent years I believe it is time to take a serious look at using flywheels in individual homes.
An additional consideration is that as decentralized power systems such as solar roofs become more widely accepted, and utility intermediate- and peak-power sales are reduced, utilities are having to think about getting into the solar energy and energy storage businesses, as is already happening in Germany. I expect this to happen in the U.S. as well.
Using Flywheels to Supply Residential Electricity Demand (July 2010)
Flywheels have always appealed to me as an interesting and potentially widely useful energy storage technology. For many years I have thought about using flywheels at individual homes to supply residential electricity demand during waking hours, using less expensive utility electricity at night to recharge the flywheel (i.e., get it up to maximum rotational speed and stored energy). Limitations have been the physical stresses on flywheel components at the high rotational speeds needed to store appreciable amounts of energy (i.e., tens of kWh) and cost. The use of advanced carbon-fiber materials may now have addressed the first limitation, and cost reductions will be associated with large scale manufacturing of the devices (still to come). The purpose of this note is to explore the feasibility and stimulate discussion of such an approach ( a few others have discussed this possibility as well), which has the potential to reduce utility peak power demands, reduce consumer costs by taking consumers off the grid at peak periods, and transform the nature of utilities. It is offered as a personal thought and does not reflect my responsibilities at the U.S. Department of Energy (2013 note: from which I am now retired).
I start by looking at residential consumer demand. According to the U.S. Department of Energy’s Energy Information Administration: “In 2008, the average annual electricity consumption for a U.S. residential utility customer was 11,040 kWh, an average of 920 kilowatt-hours (kWh) per month. Tennessee had the highest annual consumption at 15,624 kWh and Maine the lowest at 6,252 kWh.” This corresponds to an average daily demand of 11,400/365 = 31.2 kWh. Flywheels that can store 25 kWh are commercially available today (see www.beaconpower.com), and it is not unreasonable to assume that slightly larger flywheels could be easily manufactured. Thus, the idea of a flywheel providing a residence’s daily electricity demand is not unreasonable.
How do flywheels work? To quote from the Beacon Power website (there are other flywheel manufacturers as well): “Flywheel energy storage works by accelerating a cylindrical assembly called a rotor (flywheel) to a very high speed and maintaining the energy in the system as rotational energy. The energy is converted back by slowing down the flywheel. The flywheel system itself is a kinetic, or mechanical battery, spinning at very high speeds to store energy that is instantly available when needed.
At the core of Beacon’s flywheel is a carbon-fiber composite rim, supported by a metal hub and shaft and with a motor/generator mounted on the shaft. Together the rim, hub, shaft and motor/generator assembly form the rotor. When charging (or absorbing energy), the flywheel’s motor acts like a load and draws power from the grid to accelerate the rotor to a higher speed. When discharging, the motor is switched into generator mode, and the inertial energy of the rotor drives the generator which, in turn, creates electricity that is then injected back into the grid. Multiple flywheels may be connected together to provide various megawatt-level power capacities. Performance is measured in energy units – kilowatt-hours (kWh) or megawatt-hours (MWh), indicating the amount of energy available over a given period of time.
Beacon’s Smart Energy 25 flywheel has a high-performance rotor assembly that is sealed in a vacuum chamber and spins between 8,000 and 16,000 rpm. At 16,000 rpm the flywheel can store and deliver 25 kWh of extractable energy. At 16,000 rpm, the surface speed of the rim would be approximately Mach 2 – or about 1500 mph – if it were operated in normal atmosphere. At that speed the rim must be enclosed in a high vacuum to reduce friction and energy losses. To reduce losses even further, the rotor is levitated with a combination of permanent magnets and an electromagnetic bearing.”
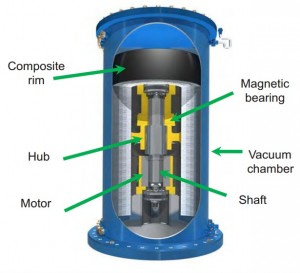
An obvious issue associated with flywheels is catastrophic failure. With rotors moving at high rotational speeds and the flywheel structure experiencing large physical stresses, what would happen if a flywheel flew apart? The industry’s answer is that they’re designed for safety, which is probably correct, but people will need additional reassurance, at least for a while. Thus, my proposal would be to place the flywheel unit under garage or carport concrete floors with a removable protective cover, to allow maintenance as needed. Flywheels can also be shielded in other “containers” as well.
Issue #2 is how long does it take to charge up a flywheel at night from full discharge? First we note that there is an energy loss associated with charging/discharging flywheels, and round-trip efficiencies have routinely been quoted in the 70-85 percent range. Recent literature quotes over 90 percent, and for purposes of calculation I shall assume 85 percent as a reasonable number to start with. Thus, to have 31.2 kWh available for useful discharge we will have to supply 31.2/0.85 = 36.7 kWh to the flywheel. A dedicated 40-amp 220 volt circuit provides power at 8.8kW. Thus, fully charging the flywheel from full discharge would require a little more than 4 hours, and this power would be purchased at low overnight rates when utility demand is lowest (at least at present). This could all change, obviously, if charging of hybrid-electric and electric vehicles, and flywheels, becomes widely used. In any case, overnight rates should be lower than daytime rates, especially peak rates.
To utilize a flywheel generator for a home a reliable control system will be required. Much design effort is going into control systems at present (e.g., for hybrid electric vehicles and smart grids), and this application would benefit from these efforts, but it would be an extra cost for the residential customer. Such costs, in addition to the cost of the flywheel and its enclosure and related electrical costs, would have to be balanced against the savings from using cheaper electricity at night. An important counterbalance is the potential set of savings to the utilities of reduced peak demand and the savings from using their currently underutilized generating equipment more fully at night. This raises the possibility of a utility advancing the costs of a flywheel system to its customers, based on its long term savings, as was done with customer installation of ground source heat pumps that also reduced utility peak demand. The advance is then paid back to the utility as an additional charge on the customer’s bill that is reduced by the use of the flywheel.
These are just initial thoughts that I hope will stimulate lots of additional thoughts and reactions. I await your feedback.
(Note: this blog was re-published in the August-September 2013 issue of The Alternative Energy eMagazine, which can be found at http://altenergymag.com/emagazine.php)